The interest in personalised treatments in the cancer arena continues to grow apace, as evidenced by the recent focus on CAR T-cell therapies. Immunotherapies like CAR T have huge potential advantages over the traditional blunderbuss-style chemotherapy and radiotherapy treatments that have dominated cancer regimens for decades, and even the more targeted kinase-based therapies that have been reaching the market in recent years. They are designed to use the patient’s own immune system to fight off the cancer.
CAR T-cells, or chimeric antigen receptor T-cells, are T-cells that have been engineered to have specific receptors that enable them to precisely target cancer cells. The T-cells are harvested and the receptor for their cancer is grafted onto the cells. The cells are then administered to the patient, ready to home in on the cancer cells, and multiply in the presence of the antigen to increase cancer-killing potential.
CAR T-cells can be constructed in a variety of ways using different protein domains
CAR T-cells can be constructed in a variety of ways using different protein domains. Most commonly, they have an extracellular domain designed to recognise the target antigen on the tumour cell, which is usually antibody-derived. This is linked to the cell’s transmembrane domain via a suitable spacer, and is in turn attached to an intracellular domain. This activates the cell when that target antigen has been recognised. The intracellular domain also often incorporates further co-stimulatory proteins to improve the response and durability of the T-cells. In this way, the CAR T-cells are engineered to seek out and destroy tumour cells.
T-cells are a vital part of the immune system. T-cell antigen receptors can detect ‘invaders’ during an infection, and activate the cells to kick off an immune response to fight them off, either killing infected cells or interacting with other cells in the immune cascade. However, if the infection or cancer cell is resistant to this form of attack, the T-cells cannot do their job. Adding the CAR to the cells can modify the cells’ response, allowing them to recognise and kill cancer cells, or inactivate a gene that is hindering the immune response.
Early – and thus furthest advanced – CAR T technology involves the collection of T-cells from individual patients, which are then modified before being returned to the patient. This autologous process has the advantage of avoiding the cells being rejected by the immune system as foreign. However, it means an off-the-shelf product is not possible, and therefore allogeneic T-cells derived from donors modified to evade rejection are now also being developed.
Various biotech companies have emerged with technologies designed to make and exploit different forms of CAR T-cells
The technology to engineer T-cells in this way dates back to the 1980s in the lab of Zelig Eshhar at the Weizmann Institute in Israel, and by the end of that decade functional CAR T-cells had been created. Since then, various biotech companies have emerged with technologies designed to make and exploit different forms of CAR T-cells, and recently there has been a surge in interest from Big Pharma in working with them.
For example, in January Amgen announced a collaboration with fellow Los Angeles-area biotech Kite Pharma. Kite has developed an engineered autologous cell therapy, or eACT, T ceplatform, and the idea behind the collaboration is to use this to develop treatments based on Amgen’s large collection of cancer targets. Preclinical work will continue to be carried out by Kite, as well as cell manufacturing and processing, up to IND filing. Both companies will then develop and commercialise their own products, including those created by Kite in partnership with the US National Cancer Institute (NCI) via a co-operative research and development agreement.
Genetic engineering
The technology is based on the genetic engineering of T-cells using a retroviral vector so that they express CAR or T-cell receptors (TCRs), both of which include extracellular tumour antigen recognition domains, and endogenous intracellular T-cell activating domains. This broad coverage enables both intracellular and membrane targets to be addressed, and there is the potential to treat both solid tumours and blood cancers.
The CAR consists of a single chain antibody domain that is designed to bind to an antigen on the surface of a tumour cell, which is linked to intracellular T-cell activating domains. Meanwhile, the TCR will recognise tumour antigen epitopes that are presented by the major histocompatibility complex, or MHC, on the tumour cell, as well as T-cell activating domains. In contrast to CARs, which recognise antigens expressed only on the cellular membrane, TCRs will recognise tumour antigens regardless of where in or on the cell they have been expressed.
A number of early stage clinical trials have been carried out at the NCI, with objective clinical responses that are both significant and durable, having been achieved in patients with a variety of metastatic diseases. These include leukaemias and lymphomas, plus melanoma and sarcoma. Multi-centre clinical trials for the lead product, CD19 CAR, in refractory diffuse large B-cell lymphoma, are now underway, and the company anticipates this product will also have potential in other B-cell malignancies.
Another product, KTE-C19 CAR, is being investigated in Phase I trials in non-Hodgkin lymphoma, with further studies planned in chronic lymphocytic and acute lymphoblastic leukaemias, and mantle cell lymphoma.
Meanwhile, on the TCR side, NY-ESO-1 TCR is being investigated in non-melanoma solid tumours that express ESO-1 receptors. Trials are at an earlier stage in TCRs to treat cervical and head and neck cancers, and a range of other tumours. Kite’s TCR capabilities were expanded in March through the acquisition of the Dutch company T-Cell Factory and its TCR-GENErator technology.
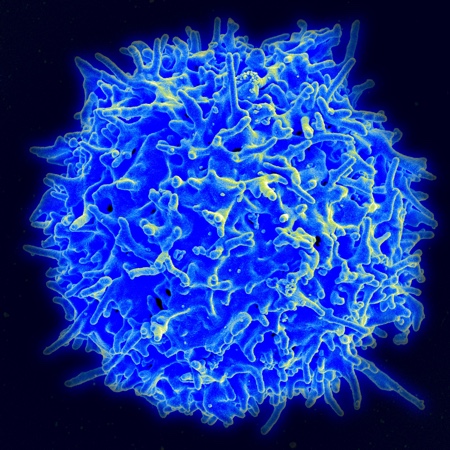
Scanning electron micrograph of a human T lymphocyte (also called a T-cell) from the immune system of a healthy donor
Credit: NIAID
Seattle-based Juno Therapeutics is also working on both CAR T-cells and T-cell receptors, and has research collaborations with three research institutes – Fred Hutchinson Cancer Research Center in Seattle, the Seattle Children’s Research Institute, and Memorial Sloan-Kettering Cancer Center in New York City. Its CAR T-cells are generally constructed using a single-chain variable fragment, or scFv, to recognise the specific antigen on a tumour cell; it is derived from a fragment of an antibody known to target that antigen. The spacer can be varied to optimise the CAR T-cell’s potency, depending on how large the antigen is, where on the antigen the scFv binds, and both the size and affinity of the scFv itself. When expressed on the surface of the T-cell, this triggers the activation of the T-cell.
The company’s furthest advanced development programmes target CD19 cell surface proteins, with four early stage clinical trials underway. A Phase I/II trial is also being carried out on WT1-specific T-cells in acute myeloid leukaemia, myelodysplastic syndrome and chronic myelogenous leukaemia. Preclinical studies are underway for cells that target L1CAM in neuroblastoma, lung, pancreatic and ovarian cancers; ROR-1 in lymphocytic leukaemia and mantle cell lymphoma; and a MUC-16/IL-12 product candidate in ovarian cancer.
In addition, the technology includes a CD28 or 4-1BB costimulatory signalling domain. By mimicking a second signal in this way, the activation of the CAR T-cells is amplified, enhancing their ability to multiply and kill the cancer cells. This way, it could be possible to engineer a T-cell that would be activated by one antigen, but inhibited if a second specific protein is present. Alternatively, the second recognition event could be required for full activation. These strategies could be applied to further increase the specificity of the T-cell for the tumour over healthy tissue.
At the end of March, Merck Serono announced an agreement with Maryland-based synthetic biology company Intrexon, to develop and commercialise CAR T-cell based therapies. Intrexon will receive an up-front payment of US$115m, and funding for the development of two targets selected by Merck Serono. It will be able to pursue further targets independently. Intrexon will carry out all development on the two selected products up until the point that they enter the clinic, and then Merck Serono will take over.
The aim of the collaboration, the companies say, is to develop products that empower the immune system to overcome the challenges currently facing CAR T therapies. Intrexon recently in-licensed additional technology from the University of Texas MD Anderson Cancer Center that will, alongside its existing proprietary technology, enable T-cells to be engineered with optimised and inducible gene expression.
The programme will take advantage of Intrexon’s RheoSwitch platform. This gene regulation technology is designed to deliver precise rheostatic control in human, animal, yeast and plant cells. It is applicable to gene therapy, biotherapeutics, and even agriculture. The idea is to give transcriptional control of a wide variety of therapeutic genes by regulating both the timing and dose of an oral activator ligand. This should allow therapeutic effects to be titrated on a patient-specific basis and in a predictable way, and it can be used as a safety switch to rapidly turn off gene expression.
Intrexon is also working with Boston-based Ziopharm Oncology, in a three-way collaboration with the University of Texas MD Anderson Cancer Center, to use RheoSwitch in CAR T-cell technology. It hopes to have five CAR products entering Phase I by the end of 2015, across a range of haematologic malignancies and solid tumours. The furthest advanced is a product directed against B-cell malignancies.
Bluebird Bio signed a deal with Celgene a couple of years ago to develop CAR T-cell based gene therapies for cancer. Working in collaboration with the Baylor College of Medicine, they are looking at them as a potential treatment for a variety of haematological malignancies and solid tumours. This programme is still in the early stages of development. Like Kite’s programmes, the technology produces autologous cells, so each patient will have to have their own cells modified before administration, rather than allogeneic cells, which are designed to evade the immune system and work in many different patients.
Another big pharma company getting into bed with a CAR T specialist biotech is Pfizer, which made a 10% equity investment in (and a large up-front payment to) Paris-based Cellectis last year. For this, and future milestone and royalty payments, Pfizer gained access to CAR T-cell therapies designed to hit up to 15 different cancer targets, plus a further 12 chosen by Cellectis where Pfizer will have first refusal on four. Preclinical development will be carried out by the two companies in collaboration for these. Cellectis will prosecute the remaining eight on its own.
Cellectis is already working with another French company, Servier, on a further six products. Among these is the potential treatment that has advanced furthest through the pipeline – UCART19 – a leukaemia therapy that targets the B-cell CD19 antigen, and the company hopes to start clinical trials with it this year. Three further products are in preclinical testing – UCART123 for acute myeloid leukaemia, and two for multiple myeloma – UCART38 and UCARTCS1.
The Cellectis process is rooted in technology based on transcription activator-like effector nucleases, or Talens. This protein-based technique uses a tailored fusion protein to reprogramme the T-cells, with the Talens acting as molecular ‘scissors’ on the cells’ DNA. It comprises a Tal effector protein that binds selectively to DNA fused to a DNA cleavage domain from the FokI bacterial endonuclease. The cells are allogeneic – they do not have to be harvested from each individual patient, instead being collected from healthy patients, and engineered so they do not attack healthy tissue. They would thus represent a ‘one size fits all’ off-the-shelf standardised cell therapy. This clearly will be cheaper and quicker than an autologous cell therapy, where new cells have to be grown for each individual patient.
Natural killer cells
Belgian cell specialist Cardio3 Biosciences acquired New Hampshire-based Celdara Medical’s OncoCyte CAR T-cell technology in January. OncoCyte uses human natural killer cell, or NK cell, receptors that target ligands present on most types of tumour. This is different from other CAR T-cell products, which typically use scFv to bind tumour antigens.
Its furthest advanced candidate, CM-CS1, uses the human NK cell receptor NKG2D, and is in Phase I trials for haematologic malignancies. The company has two further CAR T-cell programmes that target other cancer cell ligands in preclinical development. It is also working on an allogeneic T-cell platform that, like the Cellectis technology, would allow off-the-shelf therapies to be developed by creating cells that also express molecules that inhibit TCR, the molecule present on T-cell surfaces that cause rejection. The company believes this allogeneic platform will be applicable to other CAR T-cell products, too.
With any therapy that involves activating the immune system, there is a risk that over-activation can lead to serious side-effects, such as cytokine release syndrome (CRS), which can be treatment-limiting, or even fatal. CRS has resulted in several deaths in Juno’s clinical trials, and avoiding this problem is the reasoning behind its second-generation product.
Houston, Texas-based Bellicum Pharmaceuticals is using its chemical induction of dimerisation, or CID technology to try and avoid these side-effects, in collaboration with Baylor University. It enables components of the immune system to be engineered and controlled in real time. The idea is to use molecular switches – modified signalling proteins – and trigger them within the patient using a small molecule, rimiducid, in contrast to natural signalling proteins which are triggered using upstream signals in the signalling cascade. Depending on how it is engineered, it can act as a form of safety switch or an activation switch. If its CaspaCIDe technology is included, which activates caspase-9, it can be used as a safety switch. Thus far, this has been incorporated into haematopoeietic stem cell transplantation (HTSC) and TCR T-cell product candidates.
If CIDeCAR technology is engineered in, it can act as an activation switch to set the immune cells to work. These CAR T-cells include the signalling domains for the proteins MyD88 (myeloid differentiation primary response gene), and the co-stimulatory protein CD40, and also incorporate the CaspaCIDe safety signalling technology.
Either way, these switches are engineered into the immune cells, which are then returned to the patient and controlled via the administration of rimiducid by intravenous infusion. Another technology, GoCAR-T, involves T-cells modified with the company’s proprietary co-stimulatory domain MC. This includes a molecular switch that is separate from the CAR, and the CAR has no co-stimulatory domains. This enables the cells to be controlled solely through the administration (or withdrawal) of rimiducid.
Its furthest advanced T-cell product, currently in Phase I/II trials, is the CaspaCIDe based BPX-501, for HTSC. CIDeCAR product BPX-401 and CoCAR-T product BPX-601 are in preclinical development, for CD19-expressing haematological cancers and PSCA-overexpressing solid tumours, respectively.
All of these technologies remain some distance away from the market, despite all the hype that there has been around the field in recent months. There are significant hurdles still to be overcome – not least ensuring safety – but they do represent an important potential addition to the cancer-fighting arsenal.