The need for greener chemical manufacturing processes is driving research into the discovery of catalysts that provide greater selectivities using both benign reagents and non-hazardous reaction conditions. Dr Sarah Houlton reports on some recent API reaction successes.
Many structural features that are often present in APIs can prove challenging to synthesise, especially at the scale required for commercial production. While it may be possible to make them in the lab, in practice the route may involve conditions that are not conducive to scale-up, such as very high or low temperatures, or hazardous reagents. Alternative routes need to be developed, and often a metal catalyst can provide the answer to a synthetic problem by enabling a reaction to take place that would not otherwise be possible. They can be especially useful in installing stereochemistry into an otherwise achiral or racemic molecule.
Chiral tertiary alcohols are a particular problem. While they are common structural features in APIs, they are difficult to make, and the synthetic tricks such as asymmetric hydrogenation followed by dynamic kinetic resolution that can work well for secondary alcohols simply do not apply to tertiary ones. Racemic tertiary alcohols are rarely amenable to resolution, and so the stereocentre must be constructed in a selective way.
The obvious answer would be some form of chiral addition of an organometallic reagent to a ketone, and this is just what has been achieved by Minnaard’s group at the University of Groningen in the Netherlands.1 Grignard reagents are generally straightforward to prepare, but have only been successful in creating tertiary alcohols using stoichiometric amounts of a chiral ligand, and even non-asymmetric addition is difficult. Minnaard used a copper catalyst with a chiral Josiphos type chiral ligand, a system that the group had already had some success with in another unusual reaction, the enantioselective 1,2-addition of Grignards to enones.
Initial studies using CuBr•SMe2 as the metal precursor but with no ligand gave only small amounts of product in the addition of 2-ethyl-butylmagnesium to acetophenone. A screen of a range of chiral ligands suggested that Josiphos type ligands were much the most successful, giving a maximum ee of 82% in 96% yield. Subsequent reactions on a variety of substituted acetophenones were also successful, with products obtained in ees of up to 98%. Success was also achieved with differently substituted ketones, and neither the methyl nor the phenyl substitutions found in acetophenone are essential for success – various other aromatic and heteroaromatic systems worked. Different Grignards were also tried, with some success. Bulkier ones give better enantioselectivities, while methylmagnesium bromide does not work at all (Scheme 1).
Copper catalysis is also at the heart of the synthesis of another difficult moiety, the hindered aniline, from Gojko Lalic’s group at the University of Washington in Seattle.2 Again, these are common features in APIs, and while numerous couplings mediated by transition metals have been developed to make anilines, some remain difficult to make, particularly those that are hindered. Existing syntheses include going via highly reactive intermediates such as benzynes or use lithium reagents that are difficult to handle at a large scale.
Lalic looked to go via aryl boronic acid derivatives instead, because these are much more stable. As well as being readily available, they tend to have greater compatibility with a wide variety of functional groups that may already be present in the starting material. However, electrophilic amination had not been possible, and oxidative amination proved incompatible with hindered anilines.
The group started off by looking at the reaction of an aryl boronic ester with
N-benzyloxymorpholine, with IMesCuOtBu as catalyst. However, the aniline was only formed in a yield below 5%. They thought this might be as a result of the transmetalation of the aryl boronic ester being too slow, and a catalyst screen suggested that using Xantphos as the ligand might be more successful. Sure enough, in this same model system, using a catalyst prepared from (CuOtBu)4 plus the Xantphos ligand gave the aniline in a 99% yield.
However, applying this system to more hindered anilines was less successful, with a test system giving just 6% of the aniline, plus a large amount of t-butyl benzoate. Further studies indicate that this formed very rapidly. To suppress this decomposition of the electrophile they altered the reaction conditions, and found that running the reaction in the non-co-ordinating solvent toluene rather than 1,4-dioxane with lithium t-butoxide instead of the sodium salt, gave a 74% yield of hindered aniline. The best results were obtained using a concentrated reaction mixture in isooctane, with the catalyst pre-prepared in toluene.
These conditions turned out to be successful for the preparation of a wide range of hindered anilines. Heteroaromatic boronic esters can be used, and for the most part a catalyst loading of 2.5% is sufficient, although for more hindered anilines it needs to be bumped up to 5%. A wide range of electrophiles are also compatible with the reaction, including very hindered ones. Finally, replacing the lithium t-butoxide with caesium fluoride allowed a range of substrates to be coupled in good yields. (Scheme 2).
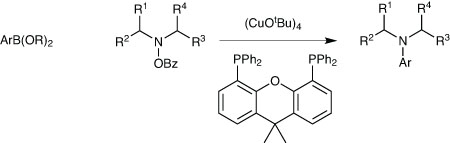
Scheme 2
The Nobel prize-winning invention of the olefin metathesis reaction has led to the ready availability of a wide range of coupled molecules, but cis olefins have remained a challenge because the catalysts tend to favour the formation of trans olefins. Now, a range of new ruthenium catalysts from Bob Grubbs’ group at CalTech has significantly improved this.3 The initial catalyst they unexpectedly identified to have cis selectivity contains an adamantyl group, which appears to be essential for the formation of the cis olefin, but other moieties on the catalyst were modified to alter its activity, notably the aryl group on the N-heterocyclic carbene and the carboxylate ligand.
Bulky carboxylates like pivalate seem to be the only types that can give the intramolecular C–H activation that is required for the catalyst to be formed, and they developed a novel route to synthesise catalyst analogues. This involved knocking off the pivalate functionality from the catalyst using sodium iodide in THF as solvent. The resulting iodo complex could then be used to make a variety of analogues by a transmetallation reaction with silver salts. Monodentate ligands could also be made in this way. Similarly, gentle steric and electronic effects were introduced into the aryl group.
The catalysts were then tested in a cross metathesis reaction – the homodimerisation of allyl benzene. Low catalyst loadings were used to facilitate the differentiation between the activities different catalysts, with the reactions carried out in THF at 35°C and a fairly high substrate concentration. The more difficult substrate methyl 10-undecenoate was then investigated with those catalysts that had performed best in the model system – those with carboxylate and nitrate substitution. Again, low loadings were used, and at 0.1% the majority still gave good conversion to the cis olefin, with the best giving conversion in excess of 90% with a short reaction time, and the best results were achieved with the nitrato substituted catalysts.
The very best performing of these catalysts were then applied to a range of more complicated reactions, notably the cross metathesis between allyl benzene and cis-1,4-diacetoxybutene. Lowering the temperature and raising substrate concentration gave a good yield of the cross metathesis product, although appreciable amounts of the diphenyl olefin were also formed with the carboxylate catalysts. The catalysts work well at low loading, with no requirement for high pressure, elevated temperature or avoidance of protic solvents, and the researchers speculate that they will find good use in the industrial synthesis of cis olefins.
hydroformulation
Alkenes can be readily hydroformylated, a process that not only creates a new carbon carbon bond, but also adds a useful functional group. However, controlling the stereo-chemistry can be more of a problem. Various catalysts are now available that allow simple substrates such as styrene to be hydro-formylated in an enantioselective fashion, but for more the complex molecules typically required as pharma intermediates and APIs, particularly branched aldehydes, control of regioselectivity is an issue. Even styrene gives typically only a 10:1 mixture when a branched aldehyde is formed. And forming branched chiral aldehydes from simple terminal alkyl olefins would be particularly useful. Work from Matthew Clarke’s group at the University of St Andrews now appears to show that this previously unachievable transformation might actually be possible.4
Previous work in the group had focused on tuning the performance of the asymmetric hydroformylation ligands Kelliphite and Ph-bpe, and they looked at creating a hybrid, non-symmetric ligand that contained the best properties of both of these ligands. Their Bobphos (best of both phosphorus ligands) was first investigated in the hydroformylation of a simple model substrate, vinyl acetate. Using Bobphos as the ligand with a rhodium catalyst gave a conversion to aldehyde in excess of 99% in 4hrs, with the reaction taking place at 2.5bar and 60°C. Only trace amounts of the linear isomer were observed, and the ee was 83%.
They then turned their attention to slightly more complex terminal alkenes, with longer sidechains. There is a good deal of demand for linear alkyl aldehydes, and many different ligands have already been investigated in the chemical industry for the hydroformylation of terminal alkenes such as 1-hexene. Almost all of these give linear selectivity, and even in those rare instances where a branched product is formed, it is not enantioselective. Even when binaphos had been tested, while it did create the branched product with decent enantioselectivity, in reality more than three-quarters of the product was actually the linear aldehyde, so the yield was impractically low.
To their surprise, Bobphos proved pretty successful in the synthesis of single enantiomer branched aldehydes. They tried a range of known hydroformylation catalysts in the reaction with allyl benzene, with the reactions carried out at sub-room temperature, 15°C, as they had already found this increases the likelihood of branched aldehyde formation. All of these were pretty unselective. But when they tried Bobphos, there was an 80% selectivity for the branched product, and the S enantiomer was formed predominantly, with an ee of 90%. Unexpectedly, neither of the two ligands from which it was derived – Kelliphos and Ph-bpe – gave any enantioselectivity at all.
Numerous other substrates that might be expected to give linear products have also been tested, and again branched aldehydes were generally formed, with a good enantioselectivity for the S enantiomer. Even allyl cyanide was successful, as was a first attempt at carrying out the reaction on a functionalised alkene.
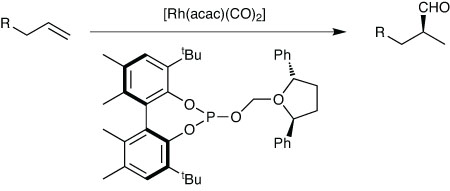
Scheme 3
Sometimes an improved catalyst can make the difference between a reaction that is practical at scale and one that is not. An example of this comes out of the labs of Takasago in Japan, where a ruthenium catalyst was developed for the hydrogenation of complex esters, for which hazardous hydride reagents are more typically used.5 The aim was to create a catalyst that would function well under mild conditions, rather than the more forcing ones of high temperature and pressure that are typically used in industrial hydrogenation processes.
Although such catalysts exist, they are less practical for industrial applications because of their overly low turnover numbers. The researchers believed this might be a result of irreversible ligand dissociation and carbonyl-ation of the ruthenium core by methanol produced in the reaction, leading to deactivation of the catalyst. If they could develop catalysts that were not carbonylated, or where the carbonylation was readily reversible back to the active catalyst, they thought they might be more effective.
a new contender
The result was a diphosphine catalyst they termed Ru-MACHO, a name they came up with because they thought the structure resembled a ‘brawny athlete holding the ruthenium’.
This is straightforward to make from [RuHCl(CO)(PPh2)] and HN(CH2CH2PPh2) 2. It has good catalytic activity, even in methanol as solvent, as it does not react with the methanol to deactivate the catalyst.
It was then used to catalyse the reduction of a range of different esters, which proceeded in the presence of sodium methoxide with good selectivity and conversion. Both aromatic and alkanoic methyl esters were reduced, as were a diester and esters containing oxygen and nitrogen atoms at the α position. Less successful were esters with β nitrogen and oxygen functionality, which did not work at all well as the substrate decomposed. In addition, the methyl ester was replaced successfully with isopropyl and t-butyl ester functionality.
On a practical level, they applied the new catalyst to the synthesis of single enantiomer 1,2-propanediol, a useful chiral building block for APIs, which can be made by the asymmetric hydrogenation of hydroxyacetone. While the turnover number of the reaction using the existing catalyst is excellent, the highest ee it can produce is 98.5%, and they wanted to make the molecule with an ee in excess of 99%, which can be achieved only by derivatisation and recrystallisation.
The alternative would be to start with methyl lactate, which is commercially available with an ee greater than 99%, and catalyse its reduction without losing chirality. They found that reaction temperature is critical – at 80°C the ee drops from 99.2% to just above 35%, but loss of chirality was minimal at 40°C.
The reaction has been carried out on a multi-tonne batch scale, using a catalyst loading of 0.05%. Starting with 2200kg of methyl lactate, and running the reaction for 12 hours at 26–28°C in methanol resulted in a 92% yield of the desired chiral diol in 99.2% ee – representing almost no loss of chirality from the starting ee of 99.6%. (Scheme 4).
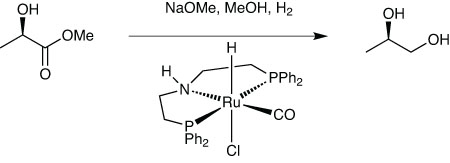
Scheme 4
Another commercial example where Ru-MACHO was successful is the synthesis of 2-(L-menthoxy)ethanol, which is currently produced by the lithal reduction of L-menthoxyacetic acid. Lithal is not ideal at a large scale because of its hazards, especially the quench at the end of the reaction to remove excess reductant. Ru-MACHO catalysed reduction removes this difficulty, and the reaction went to completion in five hours, giving an 87% yield of the desired product. These two examples show the potential of the catalyst in large scale hydrogenations, and it has now been made commercially available.
References
1. A.V.R. Madduri et al. Angew. Chem. Int. Ed. 2012, 51, 3164
2. R.P. Rucker et al. Angew. Chem. Int. Ed. 2012, 51, 3953
3. B.K. Keitz et al, J. Am. Chem. Soc. 2012, 134, 693
4. G.M. Noonan et al. Angew. Chem. Int. Ed. 2012, 51, 2477
5. W. Kuriyama et al. Org. Process. Res. Dev. 2012, 16, 166