As more therapeutic cell products are reaching the later stages of clinical trials, greater consideration is being placed on production processes that will provide commercial-scale quantities of living cell products. In particular, attention is being paid to the large-scale production of therapeutic cells,1 with downstream processing and fill-finish operations being cited as bottlenecks in current and future cell manufacturing.
Many current cell-based products are produced, frozen, stored and delivered in blood bags – technology borrowed from the blood-banking industry – owing to the available infrastructure for processing cells into bags, and for the freezing and storing of cells. While these are sufficient for small-scale processes that generate tens of product doses per lot, lot sizes of hundreds to thousands of doses will be required to supply a commercial-scale cellular product, which may preclude the use of bags.
This study evaluated the suitability of scalable final container systems in the form of stoppered vials, which are currently used for storing and distributing pharmaceutical drugs. Pharma-grade vials provide the benefits of ease-of-delivery for care givers, as well as being able to leverage existing pharmaceutical manufacturing infrastructure. Importantly, there are established standards in sizes, quality and regulatory attributes that can be drawn upon to facilitate uptake in the rapidly developing cell therapy industry.
The ideal vial-based container system should be suitable to package, store and transport cell therapy products at low temperatures, and meet pharmaceutical quality requirements to maintain cell viability over the intended shelf life. Polypropylene has been used for decades in various packaging applications, including bottles, pouches, tubes and containers, but these plastic resins have made little impact in the area of parenteral vials and prefillable syringes owing to various quality attributes.2
The use of vials has not yet been translated to cell-based biologic products
Newer plastic resins3 with glass-like clarity, lower potential extractables, various modes of sterilisation, very low moisture permeability, biocompatibility and lower particulates, have been adopted for pharma and biopharma drug delivery applications. However, the use of vials has not yet been translated to cell-based biologic products, due in part to the lack of data on the ability of cells to be successfully cryo-preserved and stored while maintaining viability and important functionalities over a reasonable shelf life, e.g. six months.
A study was devised to look at the suitability of a commercially available plastic vial. The Daikyo Crystal Zenith (CZ) was evaluated for low-temperature and cryo-preserved storage of cell systems. Such vials are currently being used to package and deliver pharmaceuticals in global markets, and are in development for biologic drugs, such as peptides, proteins and monoclonal antibodies.4–6 Being nonpolar, CZ has low moisture absorption and good drainability, which prevents water and liquid drug preparations from adhering to the vial surface. It is compatible with a wide range of pHs (2–12) and solvents, such as alcohols, ketones and Cellosolve. Its thermal characteristics enable it to withstand cryogenic temperatures as well as sterilisation by autoclaving. Compared with polypropylene, it also has lower gas and moisture permeability.14
To evaluate the suitability of CZ vials and closures for storage and transport of cell systems, three objectives were considered:
- To determine if a stem cell model system stored in these vials would maintain viability, functionality and differentiation capabilities following storage at two temperatures (–85°C and –196°C) over a six-month period;
- To determine if these vials would maintain integrity on drop testing following storage at –85°C and –196°C;
- To evaluate if filled vials would maintain container closure integrity following storage and shipment using microbial ingress and dye immersion test systems.
Three time points (one week, two months and six months); and three vial sizes (0.5mL conical, 5mL flat bottom and 30mL screw cap) were investigated. For comparison, standard 2mL screw cap polypropylene cryo-vials were used as controls.
As a stem cell model, the researchers used a mesenchymal stem cell (MSC) population isolated from human dental pulp tissue, with characteristics similar to bone marrow MSC in terms of their gene expression profiles and expression of cell surface markers.7,8 These dental pulp-derived MSCs (DPSCs) have been well characterised in the laboratory and possess the capacity to differentiate into osteoblasts, chondrocytes, adipocytes, muscle cells and neural cells.8-10
Tremendous interest has been generated in the potential use of MSCs and DPSCs for tissue regeneration and cellular therapies. Our group8,11 and others9,10 have demonstrated that DPSC can be successfully cryo-preserved and thawed with a good retention of functional viability. Owing to these properties and their availability, primary DPSC cultures were used in this study.
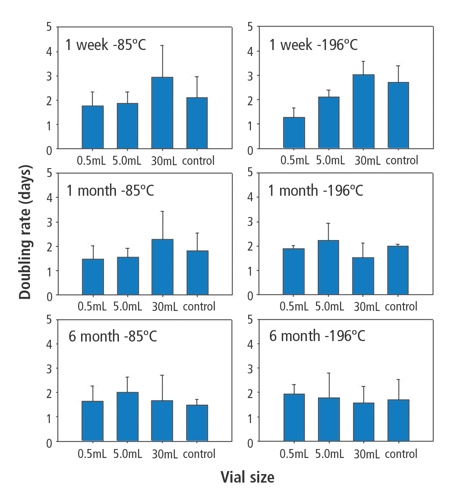
Doubling times for cells frozen in each vial type in each condition. Values are expressed as means with error bars derived from standard deviation of the means. Controls were cells frozen in standard 2mL polypropylene vials
Container integrity is a critical issue for maintaining sterility and viability of cryo-preserved cellular therapy products. With the emergence of cellular therapy as a more widely used clinical practice, improved containers and closure systems for effective safe use and cell preservation are required. While still effective for some indications, there has been more focus on the shortcomings of cryo-preserved bag storage. A study by Khuu et al. investigated a series of catastrophic bag failures first noticed in 2001.13 In that study, high rates of bag failure were associated with four specific bag lots made from poly(ethylene-co-vinyl acetate). While no serious adverse patient effects occurred, extensive bag failures led to microbial contamination of the cell product, increased product preparation time, increased antibiotic use and increased costs to replace products.
The CZ system may offer a durable and convenient alternative and avoid these potential problems. Furthermore, the pharma industry is already accustomed to the filling of various-sized vials on a large automated scale. Dental pulp-derived MSCs frozen in each type of vial exhibited excellent post-thaw viability and were able to expand readily, indicative of functional recovery. Additionally, all cultures remained free of contamination throughout the test cycle, indicating aseptic sample preparation and effective vial closure system at frozen or cryogenic temperatures. Doubling times were consistent over all vial systems, indicating comparability of the rate between fresh and stored cells in CZ vials.
However, it was noticed that one week samples had a slower doubling rate for cells frozen in 30mL vials. This is thought likely to be an artifact of the initial plating of much higher numbers of cells in the first week cultures, as lower doubling times with cells frozen in the 30mL vials were not observed with later time points that were controlled for cell number. Thawed cells retained appropriate MSC surface antigen marking and were able to undergo osteogenic, chondrogenic and adipogenic differentiation, as has been shown previously.8,11
From a mechanical standpoint, the vials exhibited excellent durability. No CZ vial exhibited gross breakage or cracking after the frozen drop test. Slight damage occurred occasionally to the flip-off caps, but did not compromise the closure integrity as demonstrated by the post-thaw dye and microbial immersion studies performed following typical transportation methods. These results, combined with the fact that none of the cell cultures became contaminated, provide good evidence that in any standard clinical application the vials provide robust container closure maintenance.
The shipping tests provided further practical evaluation of the integrity of vial systems under standard transport conditions (e.g. shipping a frozen product on dry ice) coupled with very stringent testing of the closure system following pressure cycling after receipt, storage and thaw. In these tests, all vials with a stopper seal closure system showed no evidence of dye or microbial ingress for the duration of the study and the only failures that were observed were with vials with polypropylene screw cap closures. The microbial or dye ingress in these samples may be attributed to inconsistent torque applied to the screw cap during sample preparation and/or shifting between the cap and the vial during the freeze/thaw process during expansion and contraction of materials. While this requires more investigation, these data suggest that stoppered CZ vials may give superior results to screw cap vials.
this initial proof-of-concept study indicated that the CZ vials are highly suitable for cold and cryogenic storage of biological cells
Overall, this initial proof-of-concept study indicated that the CZ vials are highly suitable for cold and cryogenic storage of biological cells, and provide a suitable container system for clinical and commercial cell therapy products. The vials were easy to freeze and more convenient to use than bags, as they could be adapted to existing storage configurations. From a practical and availability standpoint, the vials are designed with standard dimensions (13mm and 20mm openings) that are capable of fitting into traditional pharmaceutical filling lines. Additionally, CZ vials have already been approved as drug product containers in the US, Europe and Japan, and pre-sterilised versions of vials and lower temperature stoppers have been developed that may be conducive for fill-finish operations.
Compliance with compendia requirements for containers and closures used in packaging systems for cellular therapy applications should be evaluated based on the final use of the product. When evaluating compliance to specific compendia, for each application, the effect of the container closure system and the sterilisation process used should be considered. CZ vials and closures have been shown to meet the compendial requirements for plastic containers and elastomeric closures.
References
1. Kirouac DC, Zandstra PW. (2008) Cell Stem Cell 3, 369–381.
2. Akers MJ, Nail SL, Saffell-Clemmer W. (2007) TPDA J. Sci. Technol. >61(5), 337–361.
3. Eakins MN. (2005) Bioprocess Int. 3(6), 52–58.
4. Qadry SS, Roshdy TH, Char H, et al. (2003), J. Int. J. Pharm. 252, 207–212.
5. Esfandiary R, Joshi SB, Vilivalam V, Middaugh CR. Presentation at AAPS National Biotechnology Conference. Toronto, Canada, June 2008.
6. Waxman L, Vilivalam V. Presentation at AAPS National Biotechnology Conference. Seattle, USA, June 2009.
7. Shi S, Robey PG, Gronthos S. (2001), Bone 29, 532–539.
8. Perry BC, Zhou D, Xiaohua W, et al. (2008) Tissue Eng. Part C 14, 149–156.
9. Seo BM, Miura M, Sonoyama W, et al. (2005) J. Dent. Res. 841, 907–912.
10. Zhang W, Walboomers XF, Shi S, et al. (2006) Tissue Eng. 12, 2813–2823.
11. Woods EJ, Perry BC, Hockema JJ, et al. (2009) Cryobiology 59(2), 150–157.
12. Dominici M, Le Blanc K, Mueller I, et al. (2006) Cytotherapy 8, 315–317.
13. Khuu HM, Cowley H, David-Ocampo V et al. (2002) Cytotherapy 4(6), 539–549.
14. Daikyo Resin CZ Technical Report, West Pharmaceutical Services (2002).
Authors
Erik J Woods,1,2 Aniruddha Bagchi1,3 W Scott Goebel1,4 Robert Nase5 and Vinod D Vilivalam5
1. General BioTechnology, Indianapolis, US;
2. Department of Microbiology & Immunology, Indiana University School of Medicine, Indianapolis, US.
3. Department of Mech. Eng. University of Minnesota, Minneapolis, US.
4. Herman B Wells Center for Pediatric Research, Indiana University School of Medicine, Indianapolis, US.
5. West Pharmaceutical Services, Exton, US.
Daikyo Crystal Zenith is a registered trademark of (and the technology is licensed from) Daikyo Seiko, Tokyo, Japan.