The potential risks of dust cloud explosions have been known for centuries. One of the first to be reported was at a bakery in Turin in 1785 when flour was being shovelled under candlelight.1
Perhaps the most infamous dust explosion in the UK was the Bird’s Custard factory explosion in Banbury in 1981. A corn flour hopper collecting dust from expelled air overflowed to create a dust cloud that was ignited by nearby electrical equipment. The subsequent explosion literally blew the roof off the building.
The Dangerous Substances and Explosive Atmospheres Regulations (DSEAR) were published in 2002 but assessment of the area over which a dust cloud may spread — the dangerous zone — is not as well defined or as easy to calculate as it is for flammable gases and liquids.
In each case, the risk assessment starts in the same way, by considering all flammable and explosive materials (solid, liquid or gas). The behaviour of fluids can be reliably predicted, allowing the analysis of all possible scenarios in which a flammable atmosphere may be generated, and the regulations provide guidance documentation that defines the release characteristics, leading to a recommended hazardous distance (Figure 1).
Figure 1: Hazardous area distances versus release characteristics
The potential for dust clouds to explode is affected by many criteria, including particle size, moisture content, the energy required for ignition, propagation conditions, maximum overpressure, the dust class and concentration. The means that the extent of the hazardous zone is much less well defined and no equivalent hazardous distance graph can be produced.
Any assessment to ensure compliance with DSEAR guidelines must consider the worst-case release scenario and associated defined zones that determine the extent of ATmosphere EXplosible (ATEX) equipment.
This is no easy task as each scenario will be unique to that location, project, system and environment. It is not reasonable to expect the air flow in one building to be the same as another when considering airborne dusts, which is why there is no dust equivalent of the “release characteristic.”
In some cases, however, high level indicators may demonstrate that expensive dust cloud modelling is not required to define the hazardous zone limits. The following sections examine possible indicators for dust cloud risk.
Indicators
Having assessed what flammable, combustible and/or explosive materials are present and the scenarios for the potential loss of control and release, the following parameters should be assessed for dusts.
The minimum ignition energy (MIE): MIE is one of the easiest indicators of whether a powder can cause an explosive atmosphere. The lower the MIE, the higher the risk of explosion, as a very small energy input can trigger a dust cloud explosion.
For an MIE less than 3 mJ, this requires highly specific measures as a dust cloud of this powder is extremely sensitive to ignition. Between 3 and 1000 mJ requires assessment and some level of mitigation is likely to be required. Measures include grounding to prevent static electricity and the use of ATEX rated equipment.
Dust cloud generation: A study by Ansart, et al., indicates that almost any dry, free-falling flow of powder has the potential and indeed is likely to generate a dust cloud.2
However, the stability of a dust cloud is dependent on the particle size and the air movements; the worst-case dust cloud generation is for those comprising small particles. For example
- a 1µm geometric mean diameter particle requires about 13 hours to fall 1.6 m through air
- or a 0.1 µm particle, the Brownian displacement is about 15 times greater than that of the settling velocity and so the dust will remain permanently suspended if the air is still
- for particles of a mean diameter of 100 µm, the terminal velocity is approximately 1 m/s.
Thus, the particle size can often be used to provide an indication of the extent of a hazardous zone. Commonly, a particle size of 500 µm or greater is not considered to form a dust cloud but to settle out almost immediately. Care should always be given to account for ventilation air movements.
Explosion severity characteristics: MIE and particle size may provide a quick guide to rule out the possibility of explosive dust cloud formation, but the assessment is rarely conclusive. A more difficult and more subjective approach is to test or assess the dust deflagration index (KST), which is simply known as the dust constant.
The severity of the risk of combustible dust can be evaluated in an explosion classification test, which considers if the dust or powders will explode or not when scattered as a cloud. KST measures the relative explosion severity compared with other dusts.
The larger the value for KST, the more severe the explosion. KST provides the best “single number” estimate of the anticipated behaviour of a dust deflagration. The dust explosibility is classified under its KST value and is derived from the maximum rates of pressure rise, (dP/dT)max and a function of the explosion chamber volume. The equation is called the cube root law as shown here: KST = (dP/dT )max x V1/3
It should be noted that the KST value varies depending on the dynamic state of the dust cloud; that is, whether it is turbulent or quiescent, and its combustion rate. A higher-level grouped classification is the Dust Explosion Class or ST — from the German word for dust: Staub — as shown in Table I.
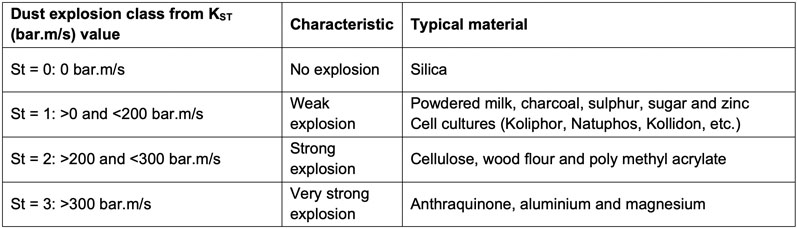
Table I: Examples of KST values for different types of dusts
Risk assessment regarding the explosive nature of a dust cloud
Table II is based on the findings of The EU RASE Project (No. SMT4-CT97-2169) and provides guidance on intolerable or acceptable risk.
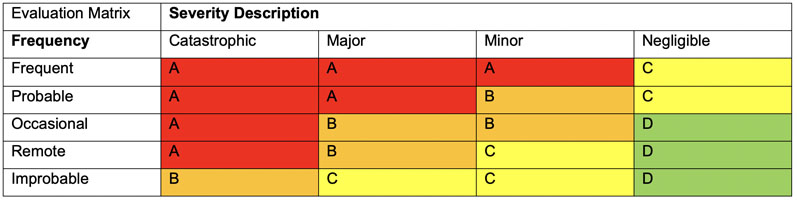
Table II: Evaluation matrix guide on acceptable risk levels
The methodology is simple: a risk evaluation should be done to determine if risk reduction is required or whether safety has been achieved. It is evident that if the estimation results in a risk level of A, it’s too high (intolerable) and additional risk reduction measures are required. Similarly, a risk level of D can be considered to be acceptable and no further risk reduction is required.
Risk levels B and C can be classed as intermediate and will normally require some form of risk reduction measures to make the situation acceptable. However, the degree of these measures will be smaller and, in the case of a risk level C, organisational reduction measures such as procedural cleaning will often be sufficient. To obtain the risk evaluation, both the severity and frequency need to be assessed.
To assess the frequency, data derived from the MIE, particle size, moisture content and cloud forming scenario can be used. For the severity, the assessment of KST and the likely outcome of an explosion are required.
In any assessment of severity, it should be considered that an explosion will happen even if the risk is extremely low. This effectively results in an overpressure or Pmax linked to the KST as per the cube root law. From the overpressure, an assessment of “damage” or severity can be made. Table III shows that a critical tipping point is approximately two bar overpressure when injury to personnel changes from severe to fatal.
Table III: Overpressure and associated biological and structural damage
Significant work has been done to link the class of a dust to overpressure and KST. A good example can be found in the American code NFPA 68, which suggests a Pmax figure — the maximum amount of pressure that explosive ignition can produce— for a given dust classification. Adjustments to Pmax can be made by considering moisture content and the dust concentration.
From the work of Huang, et al., we know that moisture content affects Pmax.3 For example, it is known that at a very low moisture content, the overpressure seen for organic dusts does not reach Pmax, restricting it to no more than 50%.
The concentration of dust per m³ of air at which Pmax occurs is the most explosion concentration; for ST1 dusts, for example, this is approximately 500–1000 g/m³.
As a guide, the level at which vision starts to be restricted for say a 100 mm particle is taken to be in the range of 50–100 mg/m³. If you can see through it, the dust concentration is low. So, for an ST1 dust in a dry environment, the overpressure becomes
Overpressure (Po) = 10 barg x 50% (moisture) x 40% (concentration) = 2 barg
Figure 2: Deflagration index with concentration for several dusts4
As such, if you can make the frequency of occurrence “improbable” and can demonstrate an overpressure of less than 2 bar — a severity of less than catastrophic — then the risk falls to within bands C or D, meaning that organisational risk reduction measures should be sufficient.
Conclusion
Although the aforementioned indicators and assessments can provide an order of magnitude of risk if your systems involve a powder with a small particle size, a low MIE and an adverse scenario that results in a high dust concentration, it is clear that procedural systems will not be adequate.
Furthermore, if in any doubt, the precautionary principle dictates that conservative mitigation must prevail.
This is why, in the case of dust systems, the hazardous zones are nearly always extended to the room limits as it is too uncertain (or expensive) to define with accuracy how far a dust cloud could travel before finding an ignition source.
Many of WHP’s clients require the use of powders, whether pharmaceutical ingredients, fermentation feeds (sugars) or foodstuffs; as such, WHP has a wealth of experience in terms of defining bespoke DSEAR requirements for powders.
The high-level indicators discussed, however, can provide a quick indication of the magnitude of risk for a given powder.
References
- D. Leith, A. Plinke and M. Boundy, "Dust Generation from Handling Powders in Industry," Am. Ind. Hyg. Assoc. 56(3), 251–257 (1995).
- R. Ansart, et al., "Dust Emissions in Powder Handling: Free Falling Particle Plume Characterisation," Chemical Engineering Journal 152(2–3), 415–420 (2009).
- C. Huang, et al., "Explosion Characteristics of Biomass Dust – Comparison Between Experimental Test Results and Literature Data," ISBN 978-5-7422-6496-7.
- W. Bartknecht, “Explosions: Course, Prevention, Protection,” Springer-Verlag, New York, NY, US (1981).