In the chiral synthesis of ingredients, finding the best bio-catalyst is often slow and costly. Paolo Braiuca, Johnson Matthey Catalysts, introduces Colour Tag Protein (CTP) technology that optimises the development and production of such enzymes.
The market requirement for chiral compounds is increasing and biotech approaches to their synthesis are emerging as one of the techniques of choice in the pharmaceutical and fine chemicals industry. The use of enzymes, such as alcohol dehydrogenases (ADH) for the synthesis of chiral alcohols, is now established and often outperforms alternative methods in terms of productivity and chiral purity. Enzyme production and engineering is, therefore, of increasing relevance to modern chemical synthesis.
Developing an enzymatic process is generally a multi-step procedure (see Figure 1). It starts with the screening of enzyme variants or microbiological strains libraries to identify the best candidate for the reaction of interest. The next steps involve the process development and development of an efficient fermentative process for enzyme production. Often during process optimisation, the characteristics of the enzyme must be fine-tuned via enzyme engineering to meet the process requirements. These steps are critical, as the economics of the final synthetic route will strongly depend on the enzyme’s catalytic performance and on its production cost. The optimisation of enzyme characteristics and of enzyme production is, therefore, of great importance.
The workflow is generally complicated by strict time frames and cost constraints, making the development of smart approaches that reduce time and costs essential for competitive advantage within the market.
Enzyme engineering is a highly inefficient procedure. Even when combining rational and random mutagenesis, the process translates into the generation of hundreds or thousands of enzyme variants. Of these 99% will be within the range of totally inefficient to exhibiting no significant improvement over the wild type enzyme. For example a large proportion of the variants will be inactive due to incorrect folding or will form inclusion bodies in the expression host yielding no viable protein. Thus current enzyme engineering research is an area where smarter techniques would be of considerable value.
Development of fermentative processes can also be extremely time-consuming. Common strategies to improve protein production include: promoter optimisation, optimisation of growth parameters and random mutagenesis. In total more than 12 separate parameters are available for optimisation in the search for an acceptable fermentation process.
It is clear that any strategy that reduces the number of experiments, speeds up the analysis, reduces development costs and increases the chance of success would be welcome.
The concept of the Colour-TAG-Protein (CTP) was designed to meet these specific needs. It finds application in enzyme engineering and enzyme production.
The CTP is an efficient marker for protein expression. It is a small protein with molecular weight lower than 15kDa, derived from one of the proteins of the cellular respiratory chain of yeasts. It is encoded by the gene ERV11 and is present in the intermembrane space of the yeast’s mitochondria. CTP is a sub-domain of its original parent enzyme, which is an oxidative biocatalyst depending on flavin adenine dinucleotide (FAD) as a co-factor.
The FAD dependence is the main determinant of interest in this enzyme, because the binding with FAD confers an intense yellow colour to the protein. CTP also had the useful property that it retained the capacity of binding FAD, while it is completely deprived of any enzymatic activity.
The primary application of CTP is as a colour-tag of fusion proteins for simple and efficient measurement of protein expression and for purification. The concept is simple; the enzyme of interest is fused by means of a short sequence containing a protease cleavage site, with the yellow CTP. CTP is small enough not to alter the folding of the enzyme and, as mentioned above, it confers an intense yellow colour to the fusion protein. As a result, the microbiological host producing the enzyme takes on a visible coloration. The colour intensity, measurable spectrophotometrically at 460nm, gives a quantification of the protein expression level.
Moreover, CTP is a distinctly polar peptide (its water solubility is 60mg/ml) able to increase the solubility of the fusion protein, thus avoiding the phenomenon of the inclusion bodies. It is stable up to 60°C, making it suitable for a wide range of fermentation conditions.
These simple characteristics are of striking importance in enzyme engineering and in optimisation of fermentative processes. With this technology, it is possible to reduce the number of variants for screening by avoiding all the mutants that would have resulted in a failure in the protein production (completely unfolded, or not-produced proteins). In addition, CTP allows a fast and direct measurement of protein expression levels in the first crude cell extract by spectroscopic methods, without the need for further purification or complex detection assays.
Host organisms, such as common Escherichia coli (E. coli), or eukaryotic cell lines, effectively produce the CTP-fused protein and the chromophore. Importantly, the formation of the FAD-complex does not depend on oxygen, which is an advantage over the well-known expression marker green fluorescent protein (GFP), a well-established method for quantifying protein expression.2 In fact, GFP and its derivates are functional only under aerobic conditions, while CTP can be flexibly applied to anaerobic conditions as well.
The generation of the CTP fusion protein is based on the so-called Colour Tag Vector, which includes the gene portion decoding for CTP, the enzyme of interest and a protease cleavage site between the two. Generally, a further histidine tag is attached at the C terminus for an easier purification of the fusion protein. A schematic representation of the vector is shown in Figure 2.
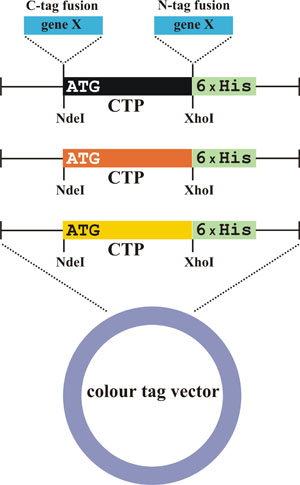
Figure 2: Schematic representation of the Colour Tag Vector. The CTP protein is shown in the three colour variants, the C-tag and N-tag fusion sequences at the C- and N-terminal of the CTP respectively are used to link the enzyme of interest and a His-tag (in green) for easier purification
A closer look at the vector reveals the use of two fusion vectors: an N-tag fusion for linking the enzyme of interest and a C-tag fusion vector used for the His-tag. It is important that the N-tag fusion vector decodes for a protease cleavage site, making separation of the CTP from the target enzyme after the development work easier, to avoid any interference of the peptide on the enzyme function at production stage.
An interesting property of CTP is that it is possible to alter its colour by mutating one single residue of its sequence. Six cysteines have been demonstrated to play an important role in the interaction with FAD. Their mutation can dramatically alter the colour of the protein by interfering with the redox equilibrium within the protein. The two most striking changes upon cysteine mutation are the black appearance of the C30S mutant and the orange colour of the C130S mutant. The UV spectrum of the mutants is completely altered, the C30S mutant revealing an additional absorbance around 580nm over the 460nm peak of the wild type, and the C130S mutant broadening the FAD peak around 460nm (Figure 3).
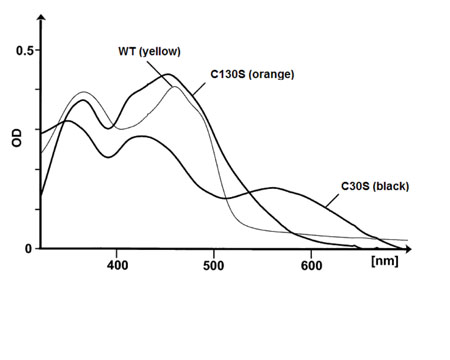
Figure 3: UV spectrum of CTP wild type, C30S mutant, and C130S mutant. Amino acid residue numbers refer to the sequence of the CTP parent protein
Structural analysis of the original protein by Hofhaus and co-workers3 revealed that the redox-active residues C130 and C133 are in close proximity to the FAD, with the C terminal cysteine (C133) being closer to the flavin structure. For the reduced central CXXC motif, where the C30 is located, an intermediate has been suggested where a thiol anion is stabilised by a charge-transfer complex with the bound FAD. Subsequently, the FAD is reduced and a disulfide bridge is formed between the cysteines.
Replacement of C130 by serine interferes with this second step and may stabilise the charge transfer complex of C133 with the FAD, resulting in the orange colour of the mutant protein. From the viewpoint of the application, three different colour tag vectors can be built, opening the possibility to clone multiple proteins in the same host, while still being able to quantitatively measure the protein expression level of each of them by a single UV-Vis spectroscopy.
In summary, the Colour Tag Protein is a well established technology platform applicable to research in enzyme engineering, protein expression and fermentation. It reduces the number of experiments significantly, cuts the time and cost of protein expression development and helps to speed up the development of enzyme production processes.
CTP currently underpins the development of the whole Johnson Matthey enzyme product line, assuring the cost efficient, eco-sustainable and high quality production of enzymes as well as effective biotransformation processes. It is currently applied to the synthesis of a range of key building blocks in the pharmaceutical and fine chemical industry.
References
1. G.Hofhaus, J.-E.Lee, I.Tews, B. Rosenberg and T.Lisowsky, Eur. J. Biochem. 270, 1528–1535 (2003)
2. G. Phillips FEMS Microbiol Lett. 204, 9-18 (2001)
3. K.L. Hoober and C. Thorpe, C. Biochemistry 38, 3211–3217 (19 99)