It brings new hope for many patients facing cancer and other diseases once thought to be incurable by equipping a patient’s cells with a means to efficiently identify and ameliorate other damaged/damaging tissues and cells within their bodies.
Driven by substantial scientific advancements in personalised therapies, investment and research in the sector has risen steeply in recent years. It is estimated that the global cell therapy market will reach $8.21 billion by 2025 — representing a compound aggregate growth rate of 14.9% from 2017, which will make it the fastest growing sector in the regenerative medicine industry.
Despite more cell therapies now being available, labour-intensive/manual processes and small batch sizes are proving to be difficult and costly roadblocks for these treatments at an industrial scale.
Cory Brown, Director of Biological Operations Technology at Zenith Technologies, A Cognizant Company, with the help of colleagues Ashish Thapa and Sylvia Diep, assesses the challenges that cell therapies present from a production perspective and explores the adoption of execution systems and their ability to help address those issues.
Cell and gene therapy
Cellular therapy (CT) is the transplantation of human cells to replace or repair damaged tissue and/or cells. CAR-T is a therapy in which receptor cells are genetically engineered to produce artificial T-cell receptors for use in immunotherapy. It enhances a patient’s own T-cells (white blood cells) to improve the immune system’s ability to identify and vigorously attack cancerous or diseased cells.
In its most simple terms, the process involves T-cells being taken from a patient’s blood via transfusion. The patient’s T-cells are then transported to a dedicated processing site, where they are mixed with a modified virus called a viral vector, which contains specially engineered genetic instructions for the patient’s cells.
These genetic instructions modify the T-cell’s own genetic instructions and provide additional instructions to grow an artificial receptor called a chimeric antigen receptor (CAR). Once expressed by the T-cells, they are transported back to a patient centre, where they are reintroduced back into the patient (transfusion).
When reintroduced to the patient, these CAR enhanced T-cells can identify and bind to distinct surface features that are unique to cancerous cells. Once bound to a cancer cell, the altered T-cell destroys and removes the cancer cell. Then, after a period, the remaining altered T-cells eventually die off themselves and are cleared from the patient.
Challenges
The high cost of such treatment is the first of a number of challenges found in cell therapies. Between either end of a supply chain — which is based on using a patient’s own biological material to formulate a product that is ultimately transfused back into the patient — are several other intense obstacles.
High production costs: Owing to the nature of the process, cell therapies are expensive. And no matter how unfortunate the reality that human health has a cost, those placed in the position to define the treatment plan will most often seek out and attempt less expensive alternatives before a patient commits or is approved for such an expensive solution.
This itself presents a set of challenges. Patients’ immune systems are often already compromised; that is, the basis of these innovative approaches is often stressed and weakened before the treatment strategy is pursued. Worse yet, these patients are often at the end of their life with only enough time remaining to receive the treatment once.
Chain of custody, transit and storage requirements: The gene therapy supply chain is often referred to as vein to vein, or V2V, which warrants additional controls and regulations. Beyond the regulations surrounding blood materials, additional expectations exist, including regulated temperature tracking during transit and storage (referred to as cold chain) and chain of custody requirements.
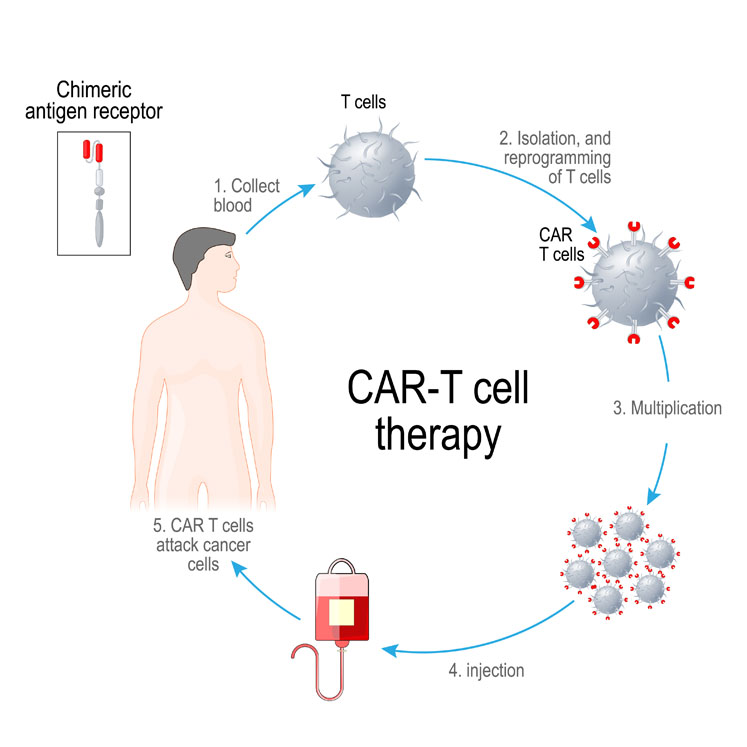
Together, these regulations detail the responsibilities required to maintain unimpugnable records for the material regarding storage locations and conditions, movements and manipulations, and all the people and equipment involved. Cell therapies are also subject to a short administration window following production, which brings further challenges.
Small batch sizes and need for flexibility: Because a unique product is produced for each patient, processing is not possible in bulk quantities. Rather, the process is more analogous to research and development or laboratory environments. Similarly, the equipment used throughout the process is designed for these other spaces … and frequently comes from manufacturers who are unfamiliar with GxP expectations.
In addition, there is often the need to adapt processes to suit each unique product, requiring a flexible manufacturing set-up. This leaves opportunity for new development as the industry emerges, but it also leaves many disparate technologies that do not integrate or function as a single system. These are challenges not often seen in larger biological bulk manufacturing.
Stringent QA requirements: Additionally, well warranted evidence from quality control organisations must be provided. To that end, multiple tests can take in excess of a month to generate results. These tests must be completed to provide assurances that the product is what was intended to be manufactured and must be delivered from laboratories capable of commensurately providing those assurances.
Applying execution systems (ES) to address challenges and improve processes
The long-term success of cell therapies is dependent on not only proving that they can produce the desired biological response, but also finding more innovative ways to produce these complex products. To facilitate the more widespread adoption of cell therapies as a viable treatment plan, costs must be reduced … and patient/consumer confidence must be resolute.
The adoption of ES and its integration with equipment and other business systems appears to be the simplest way to address both needs.
Reducing production costs: Implementing ES, including manufacturing execution systems (MES) and laboratory execution systems (LES), can reduce production costs while maintaining quality assurance.
By increasing product consistency and repeatability through standardisation and streamlined workflows, these systems can reduce process deviations and the requirement for rework and exceptions (errors and events), while also ensuring documentation time is kept to a minimum. A “right first time” approach, with alerts for certain parameters or other escalation points, can also contribute to cost-efficiency improvements.
Increasing quality assurance: The enforced compliance that is synonymous with ES provides reassurance that the right data is logged for quality purposes. This also eliminates the requirement for paper-based batch records, delivering a considerable ROI and meaning that less resources and manual processes are necessary.
ES also presents the opportunity to apply the “don’t repeat yourself” (DRY) principle aimed at reducing repetition and enabling a single change to be replicated across multiple systems.
Improving supply chain custody and cold storage: Enhancements to the tracking of supply chain custody are another advantage of ES adoption, with a clear history being available that documents who owns the material (past/present), the status of the material, who has responsibility for completed operations, the tracking of sample movement and the documentation of results.
Tracing of cold storage conditions and treatment of material, as well as thaw cycle tracking is also simplified. These capabilities once again help to bring enforced compliance and improvements to the integrity of data surrounding materials history.
Enforcing compliance capabilities and standardisation of batches: Challenges associated with the short administration window of cell therapies can also be overcome through the enforced compliance capabilities of ES.
By using a review-by-exception concept, the time required to identify, review and resolve process exceptions during manufacturing processes can be reduced, enabling shorter timelines to product release and getting products to patients faster. Small batch sizes can also be standardised, with an ES following a defined set of steps with computer control for every batch, ensuring any deviations can be easily identified.
Minimising ill-fitting equipment and non-integrated technologies: The impact of ill-fitting equipment and non-integrated technologies is also being minimised with ES by bringing in better procedural controls around equipment, including good automated manufacturing practice (GAMP) requirements for classification of computerised systems.
New equipment is now also emerging from development laboratories that may offer a lower risk profile. As expectations grow around cell therapies and the promise they hold, implementing ES and integrating workflows with existing equipment offers significant value and will be important in delivering these treatments to more patients.
The future of cell and gene therapy
Although few patients are currently treated with gene therapy regimens compared with other treatment options, the market is quickly growing. The recent entrance of major biopharmaceutical companies into this sector is anticipated to drive increased investment and manufacturing efficiencies of these therapies.
The successful growth of the industry will largely depend on transforming the manufacturing process from open and manual to closed and automated.
This transformation will result in reduced contamination risk, increased product consistency, improved data integrity and better chain-of-custody traceability.
Execution systems, including MES and LES, have the potential to play a critical role in this transformation by delivering an end-to-end integrated platform for manufacturing. The introduction of execution system technologies can facilitate scale-up, digitisation and provide insight to optimise gene therapy processes.
Once recognised, these efficiencies will lead to an increased adoption of execution platforms within the sector, enabling the delivery of breakthrough cancer therapies to millions of new patients globally. Establishing reliable and consistently capable processes will prove to be the key to successfully and profitably producing cell therapy solutions.